BILL WICKNER LAB at Dartmouth
Vacuole Membrane Fusion
1984 to the present
MEMBRANE FUSION DISCOVERIES OF THE LAB
Spatially & cell-cycle controlled vacuole inheritance & fusion- Weisman
VAC genes & mutants- Weisman, Shaw
Cell-free vacuole fusion- Conradt, Haas
SNAREs of vacuole fusion, in cis and in trans- Haas, Ungermann, Collins
Rab GTPase, Sec17, Sec18- Haas
ATP-dependent SNARE disassembly, releasing Sec17- Mayer, Haas, Ungermann
Priming triggers tethering- Mayer
Microdomain of membrane fusion proteins & lipids- Wang, Merz, Fratti
HOPS complex, and its isolation- Seals, Stroupe, Hickey
Isolation of prenylated Rab- Stroupe
HOPS binds 2 Rabs, each SNARE, phosphoinositides, and Sec17- Stroupe, Zick, Orr, Song
Reconstitution of fusion with pure components- Sato, Mima, Stroupe, Zick
Sec17 and Sec18 promote HOPS-dependent fusion- Mima, Xu, Zick, Song
HOPS catalyzes 4-SNARE assembly- Orr, Torng
SNAREs bind Sec17 or HOPS but not both- Collins
And later, by lab alumni in their own labs
HOPS subunit connectivity and HOPS structure- Merz, Ungermann
Sec17 bypass of partial SNARE zippering- Merz
Ccz1/Mon1 is essential as the GEF- Weisman, Klionsky, Ungermann
CORVET, the endosome's HOPS- Ungermann
Vam7 cycling- Ungermann
The origin of our lab's study of membrane fusion was when Jeff Schatz and I sat at a cafe outside the Basel, Switzerland train station (when Randy and I were on sabbatical there), awaiting an arriving seminar speaker. "Well Jeff," I said, "Mitochondria used to be studied for ox phos, and now [thanks to you and Walter] for protein import-- what's the next big topic for this organelle?" Jeff immediately replied "Why their shape, their position in the cell, their division and fusion, and their inheritance during cell division, of course." I saw the clouds part, beams of light shown down from Heaven, and Cherubim and Seraphim were playing their harps at this divine outpouring of insight. "Wow," I said in my finest American English. "That's fantastic*. Are you going to study that?" "No" said Jeff. "I'm busy studying protein import". "Do you mind if I study that?" "Be my guest". This was a religious experience.
What follows here isn't a scholarly review of the membrane fusion field at all. Rather, it's a history of how my labmates and I explored membrane fusion with yeast vacuoles, focused on each labmate's contributions before heading off into the world and their own spectacular careers.
Soon after my family returned to Los Angeles post-sabbatical, Lois Weisman joined the lab to study protein translocation. After a few days, she came into my office and announced that she found our work boring!! Being Lois, she spoke with tears in her eyes and profuse apologies, but with sincerity. I told her of my epiphany with Jeff, and she immediately changed direction. Lois began with the vacuole instead of mitochondria, since they were readily labeled by FM4-64 (while mitotracker, or GFP-tagging proteins, hadn't yet appeared) and vacuoles were readily purified by flotation. She also discovered that ade2 mutant yeast have an intense fluorescence within their vacuole, and the fluorescence remains after fluorophore production is shut off by adding adenosine to the growth medium (1). Using a standard fluorescence microscope, primitive by today's standards, Lois discovered that vacuoles promulgate a stream of vesicles at a precise point in the cell cycle, soon after bud emergence, and this stream heads directly into the bud (2).
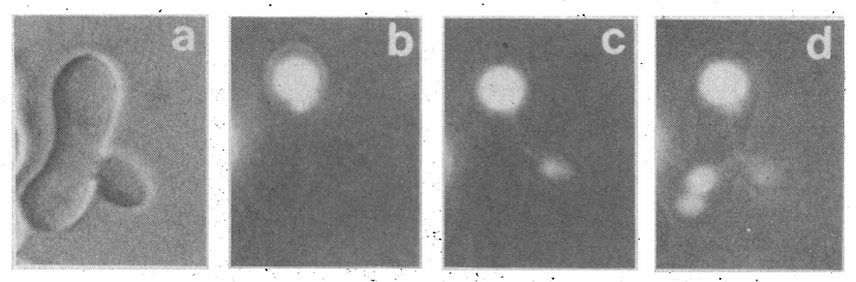
Figure 1. Ade-2 fluorophore from one vacuole in a zygote (b) streams into the bud (c) and thence to the other, previously nonfluorescent vacuole (d). From ref. 2.
In the ensuing years, in her own lab, Lois illuminated the elegant molecular mechanisms involved, in which a Vac17 adapter protein binds to both the vacuolar surface protein Vac8 and to Myo2. Myo2 moves on cellular railroad tracks of actin into the bud for organelle delivery, whence the Vac17 is degraded after arrival in the bud to render the trafficking unidirectional (3). Meanwhile, Lois began to isolate "vac" mutants which were blocked in vacuole inheritance (4), and Janet Shaw joined in this enterprise (5).
At this time Barbara Conradt appeared in my UCLA office door, a beginning graduate student a long way from home. She'd come from Germany to UMass Amherst for college, then to a lab in Biology at UCLA, but was seeking a home for her thesis work. Barbara undertook the development of an in vitro assay of vacuole inheritance, but found that vacuoles in yeast spheroplast lysates grew larger over time, reflecting fusion which could be confirmed biochemically, rather than forming structures which could be assigned to inheritance (6).
In 1993, shortly after "The Long March" across the country to Dartmouth, Albert Haas developed an amazing colorimetric assay of this vacuole:vacuole "homotypic" fusion (7). Vacuoles were purified from two strains of yeast, one lacking the lumenal Pep4 protease (and therefore accumulating the lumenally-oriented and catalytically-inert pro-Pho8 phosphatase) and the other bearing Pep4 but deleted for the PHO8 gene. Neither vacuole population had active phosphatase but, upon incubation with ATP and cytosol, the vacuoles fused, exposing the lumean proPho8 to protease which cleaved it to its mature and active form, which has a convenient colorimetric assay.
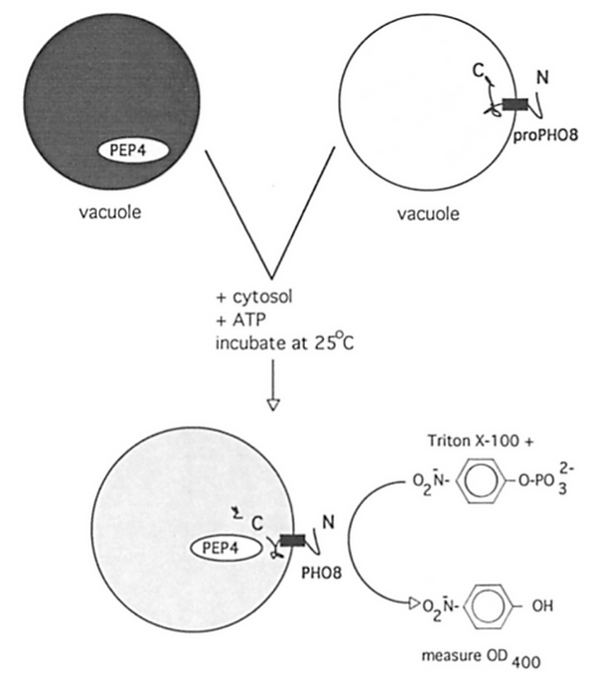
Figure 2. Assay of vacuole fusion (7).
The lab "yeasties" (Albert, Barbara, and Teresa Nicolson, soon joined by Andreas Mayer and then Christian Ungermann from Munich), could arrive at work at 8:30, then harvest, spheroplast and osmotically lyse the yeast by 10:00, then float vacuoles and assay their fusion with quantitative results, all before lunch! I've told this to prospective students, noting that "If the prospect of a quantitative, rapid simple colorimetric assay of complex biology doesn't thrill you, you may be in the wrong profession!"
This was our daily ritual- make vacuoles after breakfast, then several rounds of assays before dinner- for the next 15 years, 1993 to 2008. Collaborative studies of Albert and Christian Ungermann with Ben Nichols and Hugh Pelham allowed definition of the 4 vacuolar SNAREs (8), and demonstration that the R-SNARE (Nyv1, or "R") functions on the opposite fusion-partner membrane from the 3 Q-SNAREs, or "Qa, Qb, Qc") (9). Albert purified Sec17, Sec18, and the vacolar Rab Ypt7 and studied their functions (10). In 1996, Andreas and Albert demonstrated that vacuole fusion could be staged, either kinetically or through inhibitory antibodies (11). The first "priming" stage needs Sec17, Sec18, and ATP to release vacuolar Sec17 and to resolve the SNAREs from each other. Priming can occur on separate vacuoles, and prepares them for Ypt7-dependent tethering and SNARE pairing. Docked vesicles then slowly fused. This article had a major impact on the field, steering it away from Sec17 and Sec18 as engines of fusion and towards SNAREs; only now are Sec17 and Sec18 returning to this limelight (see below).
Meanwhile, the genetics of vacuole fusion had leapfrogged our efforts at biochemistry. Yoh Wada and colleagues simply mutagenized yeast, grew colonies on plates, and examined colonies one at a time for their vacuole morphology. Vacuoles undergo constant fusion and fission, but some lab strains have at steady state only one or two large vacuoles, easily visualized by Nomarski light microscopy. When fusion is blocked, fission proceeds unabated, and cells exhibit numerous small dot-like vacuoles instead of one or two big ones. This was termed the vam (vacuole morphology) phenotype (12). They found 9 VAM genes which were needed for the full-size organelle, and that several of these proteins constituted a large, water-soluble complex (13). We shamelessly asked Dr. Wada for his strains, and he generously sent these plus precious antibodies to each encoded protein, four of which Emr's group had also found to be in a complex (14). This enabled the Emr group (15) and Darren Seals in our lab (16) to show that six of the VAM genes, now more commonly known by their VPS (vacuole protein sorting) nomenclature of Stevens and Emr, form a complex, the Vps 11, 16, 18, 33, 39, and 41 complex, termed HOPS (HOmotypic fusion and vacuole Protein Sorting). HOPS was shown to bind to Ypt7:GTP more than Ypt7:GDP. HOPS shifts its apparent size when vacuoles are incubated with ATP and Sec18, perhaps reflecting their displacement from a disassembling SNARE complex (16). Kevin Collins (16a) showed that vacuolar SNAREs are associated with HOPS or with Sec17, but never with both,
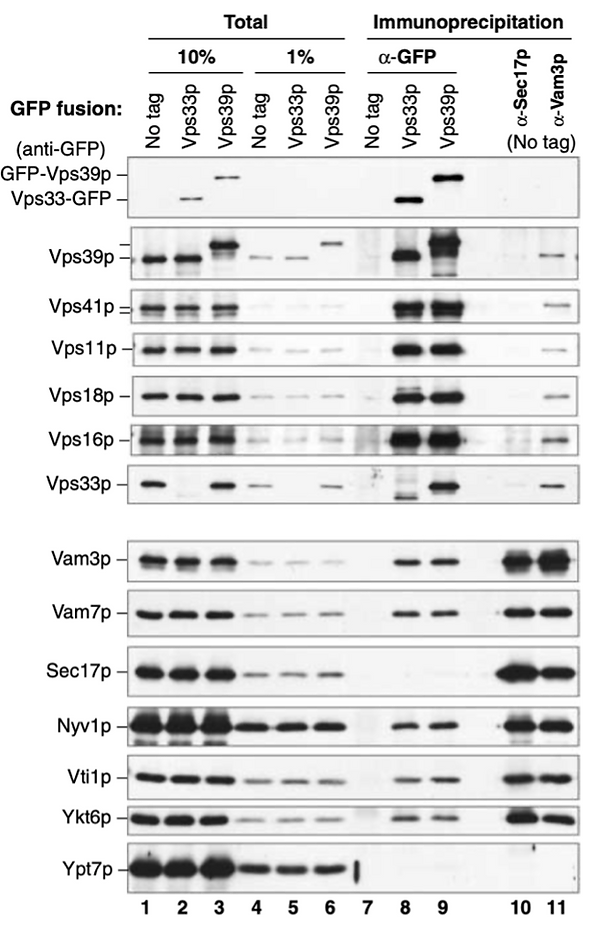
Figure 3. In extracts of purified vacuoles, the pulldown of HOPS by a GFP-tag on HOPS subunit Vps33 or Vps39 (lanes 8,9) shows association with SNAREs but not with Sec17, whereas pulldown of Sec17 (lane 10) shows association with SNAREs but not with HOPS. From (16a).
and that added Sec17 could displace HOPS from SNAREs, as later confirmed with pure proteins in the reconstituted fusion reaction (16b). Chris Stroupe tagged the Vps33 subunit of HOPS, purified the hexameric complex by this tag (17), and showed that vacuoles from a vps11 strain which were incapable of in vitro fusion were restored for fusion by the isolated HOPS. This was exceptionally courageous; the tag might have interfered with activity, the vps11-1 vacuoles might have been permanently occupied by the crippled mutant HOPS, and there was no assay to test whether the purification conditions harmed HOPS. HOPS is an amazing protein, a protein to love. It binds not only the Rab Ypt7, but each of the 4 vacuolar SNAREs, as shown by Chris (17), by collaborative studies with David Baker in Fred Hughson's group at Princeton (18), and by Amy Orr (19). HOPS also binds specific lipids including phosphoinositides (17), and even Sec17 (20). Thomas Torng showed very directly that HOPS is activated by its associations with Ypt7 and vacuolar lipids to catalyze the assembly of the four vacuolar SNAREs (21), and it was shown by Vinny Starai to be essential for "proofreading" of correct SNARE composition and structure (22). Vinny and Chris Hickey engineered a yeast strain which co-overexpresses each of the six HOPS subunits, allowing isolation of mg amounts (22, 23). Hongki Song has shown that HOPS isn't just a tether, or a tether with Sec1-Munc18 affinity for the R- and Qa-SNAREs, but can support the assemly of rapid-fusion intermediates which lack any one Q-SNARE and exhibit a "burst" of rapid fusion on its addition (25). HOPS remains a central object of fascination, and study, and perhaps even idolatry, to this day.
In 2000, Li Wang joined the lab, and looked for any spatial clustering of the proteins which mediate fusion. To this end, she created strains with GFP tags on either Ypt7, the SNAREs, and HOPS subunits. She and Alex Merz discovered that these central catalysts of fusion are clustered in a ring-shaped microdomain which surrounds the two apposed membranes of docked vacuoles, termed the "vertex ring" (26). Rudy Fratti found that key lipids, such as ergosterol and phoshoinositides, were also enriched in the vertex ring (27).

Figure 4. Certain lipids which are required for vacuole fusion are enriched at a ring-shaped microdomain surrounding closely apposed docked vacuoles, seen by their binding fluorescent lipid ligands as dots in optical cross-section. From (27).
Not only are proteins interdependent for vertex ring enrichment, the lipids depend on each other for vertex enrichment as well. Strikingly, Rudy showed that fusion proteins and fusion-essential lipids are interdependent for their microdomain enrichment. This may be a paradigm for membrane microdomains in general, needing exploration in other systems.
While the lab studied fusion of isolated vacuoles for 15 years, our goal from the start was to isolate all the important proteins and lipids and to reconstitute fusion will all-pure components, as we had done for protein translocation in 1990. In 1997, Ken Sato joined the lab for this purpose. He found that he could solubilize Pep4-deficient vacuoles in detergent, then re-form by detergent removal proteoliposomes which bore proPho8 and were able to fuse with pho8PEP4 vacuoles. Prior to reconstitution, the extract could be fractionated, and both SNARE complex and Ypt7 were essential for functional reconstitution. This amazing work (28) was our "marker" in the literature that fusion entailed more than SNAREs alone.
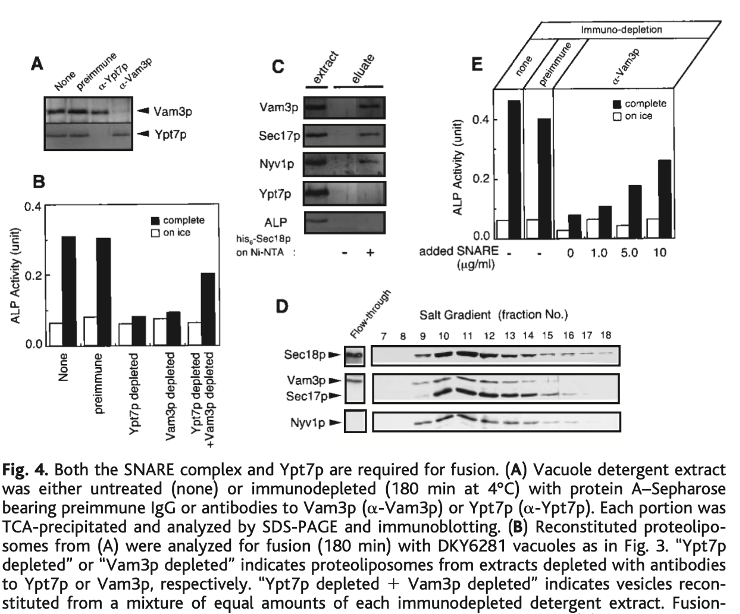
Figure 5. Detergent extracts of vacuoles could be reconstituted into proteoliposomes which would fuse with other vacuoles. Specific immunodepletion of either the Rab Ypt7 or the SNAREs (A) allowed demonstration that both immunodepleted extracts had to be mixed to reconstitute fusion-active proteoliposomes (B). The extract was fractionated, allowing partial purification of a SNARE:Sec17:Sec18 complex (D) which would complement the SNARE-immunodepleted crude extract to create active proteoliposomes (E). From (28).
Building on the Rothman group's finding (29) that vacuolar SNAREs would associate with each other and support fusion (29), Joji Mima created SNARE proteoliposomes with vacuolar lipids which fused in response to Sec17, Sec18, ATP, and HOPS from Chris Hickey (30). This breakthrough was extended by Chris Stroupe, who purified prenlylated Ypt7 from a yeast overexpression strain and found conditions where it was required for fusion (31).
At this juncture, our fusion reconstitution system still required SNAREs at a 1:1000 molar ratio to lipids, far higher than the physiological 1:32,000 levels. Paola Zucchi showed that these levels of SNAREs were causing massive proteoliposome leakage, and Michael Zick devised an elegant assay which distinguished fusion from lysis (32).

Figure 6. Proteoliposomes with lumenal Cy5-streptavidin or biotinylated phycoerythrin (a highly fluorescent protein) are prepared with recombinant Ypt7 and the four vacuolar SNAREs. They fuse rapidly and efficiently when given purified HOPS, Sec17, Sec18, and ATP (32).
Fusion could be achieved at physiological SNARE levels when the lipids had the full vacuolar head-group composition and a physiologically fluid fatty acyl composition; it was then fully dependent on the Rab, and there was little accompanying lysis (33).
All these studies set the stage for our current efforts. While Thomas has demonstrated that Sec17 will promote the completion of zippering of HOPS-assembled SNAREs (21), Hongki has shown that fusion can proceed even when each of the 3 Q-SNAREs has its C-terminal layers completely crippled for zippering (34). Sec17 and, mysteriously, Sec18 are clearly building on the platform of partially assembled SNAREs, and then contributing directly to fusion. Furthermore, Amy is studying how the Juxta (J) region of the R and Qa SNAREs, lying between the SNARE domains and the transmembrane domain, must be matched with their SNARE domains or TM domains for full function.
Major questions for the coming years include:
1. Is HOPS still engaged with the SNAREs as they complete zippering, or is it displaced by Sec17?
2. How does HOPS catalyze SNARE assembly?
3. How to develop assays of bilayer alterations during fusion itself. Content leakage?
4. Is post-fusion lysis still SNARE/HOPS/17/18-dependent?
5. Developing with split luciferase a real-time assay of SNARE assembly.
6. Isolating complexes of HOPS, Ypt7, SNAREs, 17, and 18 for stoichiometry and single-particle structures.
7. How is Sec18 promoting Sec17-dependent, zippering-independent fusion?
8. Is Sec18 ATP hydrolysis coupled to the fusion cycle?
9. Which vacuolar lipids cooperate with Ypt7 to activate HOPS for catalysis of SNARE assembly and which support fusion after the SNAREs have assembled, +/-zippering and +/-Sec17/18?
10. As the SNARE levels are lowered from 1:8k to 1:64k, does the % reliance on Sec17/18 for fusion increase? Or, with YR and YQaQb proteoliposomes, can one see this as you vary the [Qc]?
*Two old ladies, Martha and Maude, sat in the park. Martha began bragging about her son. "First he gave me a lovely coat." "Fantastic!" said Maude. "Then, tickets for a cruise around the world." "Wow, that's really Fantastic" said Maude. "And most recently, massage and manicure/pedicure every week!!" "Wow!" said Maude, "That's really FANTASTIC!" Martha paused in her bragging, and began needling her companion. "So your son, what's he done for you?" "Well" said Maude, "He's a good boy, but not that special." "Oh really?" said Martha, sensing her advantage, "What HAS he done for you, now or ever?" "Well" said Maude, "He sent me to charm school." "Well dear, that's very, very nice." said Martha, who clearly felt she'd won. "Tell me, what did you learn in charm school?" "I learned to say "fantastic" instead of bullshit." An old Racker joke, told me that same day by Jeff.
References
1. Weisman, L.S., Bacallao, R. and Wickner, W. (1987). Multiple methods of visualizing the yeast vacuole permit evaluation of its morphology and inheritance during the cell cycle. J. Cell Biol. 105, 1539-1548.
2. Weisman, L.S. and Wickner, W. (1988). Intervacuole exchange in the yeast zygote
defines a new pathway in organelle communication. Science, 241, 589-591.
3. Weisman, L.S. () Organelles on the move: insights from yeast vacuole inheritance. Nature Rev/Mol. Cell Biol. 7, 243-252.
4. Weisman, L.S., Emr, S.D., and Wickner, W.T. (1990). Mutants of Saccharomyces cerevisiae which Block Intervacuole Vesicular Traffic and Vacuole Division and Segregation. Proc. Natl. Acad. Sci. USA, 87, 1076-1080.
5. Shaw, J.M. and Wickner, W. (1991). vac2: a yeast mutant which distinguishes vacuole segregation from Golgi-to-vacuole protein targeting. EMBO J. 10, 1741-1748.
6. Conradt, B., Shaw, J., Vida, T., Emr, S.D., and Wickner, W. (1992). In vitro reactions of vacuole inheritance. J. Cell Biol. 119, 1469-1479.
7. Haas, A., Conradt, B., and Wickner, W. (1994). G-protein ligands inhibit in vitro reactions of vacuole inheritance. J. Cell Biol. 126, 87-97.
8. Nichols, B.J., Ungermann, C., Pelham, H.R.B., Wickner, W.T., and Haas, A. (1997) Homotypic vacuolar fusion mediated by t- and v-SNAREs. Nature 387, 199-202; Nichols, B.J., Ungermann, C., Pelham, H.R.B., Wickner, W.T., and Haas, A. (1997) Homotypic vacuolar fusion mediated by t- and v-SNAREs. Nature 387, 199-202.
9. Ungermann, C., Sato, K. and Wickner, W. (1998) Defining the functions of trans-SNARE pairs. Nature 396, 543-548.
10. Haas, A. and Wickner, W. (1996). Homotypic vacuole fusion requires Sec17p (yeast a-SNAP) and Sec18p (yeast NSF). EMBO J.15, 3296-3305; Haas, A., Scheglmann, D., Lazar, T., Gallwitz, D., and Wickner, W. (1995) The GTPase Ypt7p of Saccharomyces cerevisiae is required on both partner vacuoles for the homotypic fusion step of vacuole inheritance. EMBO J. 14, 5258-5270.
11. Mayer, A., Wickner, W., and Haas, A. (1996). Sec18p (NSF)-driven release of Sec17p (a-SNAP) can precede docking and fusion of yeast vacuoles. Cell 85, 83-94.
12. Wada, Y. Ohsumi, Y., and Anraku, Y. (1992) Genes for directing vacuolar morphogenesis in Saccharomyces cerevisiae. I. Isolation and characterization of two classes of vam mutants. J. Biol. Chem. 267, 18665-18670.
13. Nakamura, N., Hirata, A., Ohsumi, Y., and Wada, Y. (1997) Vam2/Vps41p and Vam6/Vps39p are components of a protein complex on the vacuolar membranes and involved in the vacuolar assembly in the yeast Saccharomyces cerevisiae. J. Biol. Chem. 272, 11344-11349.
14. Sato, T.K., Rehling, P., Peterson, M.R., and Emr, S.D. (2000) Class C Vps protein complex regulates vacuolar SNARE pairing and is required for vesicle docking/fusion. Mol. Cell 6, 661-671.
15. Wurmser, A.E., Sato, T.K., and Emr, S.D. (2000) New Component of the vacuolar class C-Vps complex couples nucleotide exchange on the Ypt7 GTPase to SNARE-dependent docking and fusion. J. Cell Biol. 151, 551-562.
16. Seals, D., Eitzen, G., Margolis, N., Wickner, W., and Price, A. (2000) A Ypt/Rab effector complex containing the Sec1 homolog Vps33p is required for homotypic vacuole fusion. Proc. Natl. Acad. Sci. USA 97, 9402-9407.
16a. Collins, K.M., Thorngren, N.L., Fratti, R.A., and Wickner, W.T. (2005) Sec17 and HOPS, in distinct SNARE complexes, mediate SNARE complex disruption or assembly for fusion. EMBO J. 24, 1775-1786.
16b. Schwartz, M.L., Nickerson, D.P., Lobingier, B.T., Plemel, R.L., Duan, M., Angers, C.G., Zick, M., and Merz, A. (2017) Sec17 (a-SNAP) and an SM-tethering complex regulate the outcome of SNARE zippering in vitro and in vivo. eLife DOI: https://doi.org/10.7554/eLife.27396.001
17. Stroupe, C., Collins, K.M., Fratti, R.A., and Wickner, W.T. (2006) Purification of active HOPS complex reveals its affinities for phosphoinositides and the SNARE Vam7p. EMBO J. 25, 1579-1589.
18. Baker, R.W., Jeffrey, P.D., Zick, M., Phillips, B.P., Wickner, W.T., and Hughson, F.M. (2015) A direct role for the Sec1/Munc18-family protein Vps33 as a template for SNARE assembly. Science 349, 1111-1114.
19. Song, H., Orr, A., Lee, M., Harner, M., and Wickner, W. (2020) HOPS recognizes each SNARE, assembling ternary trans-SNARE complexes for rapid fusion upon engagement with the 4th SNARE. eLife DOI 10.7554/eLife.53559.
20. Song, H., Torng, T., Orr, A., Brunger, A.T., and Wickner, W. (2021) Sec17/Sec18 can support membrane fusion without help from completion of SNARE pairing. eLife, under review.
21. Torng, T., Song, H. and Wickner, W. (2020) Asymmetric Rab activation of vacuolar HOPS to catalyze SNARE complex assembly. Mol. Biol. Cell, DOI: 10.1091/mbc.E20-01-0019.
22. Starai, V.J. and Wickner, W. (2008) Yeast HOPS complex proofreads the SNARE 0-layer and activates it for fusion. Mol. Biol. Cell 19, 2500-2508.
23. Hickey, C. and Wickner, W. (2010) HOPS initiates vacuole docking by tethering membranes before trans-SNARE complex assembly. Mol Biol. Cell 21, 2297-2305.
24. Song, H. and Wickner, W. (2019) Tethering guides fusion-competent trans-SNARE assembly. Proc. Natl. Acad. Sci. USA, 116, 13952-13957
25. Song, H., Orr, A., Lee, M., Harner, M., and Wickner, W. (2020) HOPS recognizes each SNARE, assembling ternary trans-SNARE complexes for rapid fusion upon engagement with the 4th SNARE. eLife DOI 10.7554/eLife.53559.
26. Wang, L., Seeley, S., Wickner, W. and Merz, A. (2002) Vacuole fusion at a ring of vertex docking sites leaves membrane fragments within the organelle. Cell 108, 357-369; Wang, L., Merz, A., Collins, K., and Wickner, W. (2003) Hierarchy of protein assembly at the vertex ring domain for yeast vacuole docking and fusion. J. Cell Biol. 160, 365-374.
27. Fratti, R., Jun, Y., Merz, A.J., Margolis, N., and Wickner, W. (2004) Interdependent assembly of specific "regulatory" lipids and membrane fusion proteins into the vertex ring domain of docked vacuoles. J. Cell Biol. 167, 1087-1098.
28. Sato, K. and Wickner, W. (1998) Functional reconstitution of Ypt7p GTPase and a purified vacuole SNARE complex. Science, 281, 700-702.
29. Fukuda, R., McNew, J.A., Weber, T., Parlati, F., Engel, T., Nickel, W., Rothman, J.E., and Söllner, T.H. (2000) Functional architecture of an intracellular membrane t-SNARE. Nature 407, 198-202
30. Mima, J., Hickey, C., Xu, H., Jun, Y, and Wickner, W. (2008) Reconstituted membrane fusion requires regulatory lipids, SNAREs and synergistic SNARE chaperones. EMBO J. 27, 2031-2042.
31. Stroupe, C., Hickey, C.M., Mima, J., Burfeind, A.S. and Wickner, W. (2009) Minimal membrane docking requirements revealed by reconstitution of Rab GTPase-dependent membrane fusion from purified components. Proc. Natl. Acad. Sci. USA 106, 17,626-17,633.
32. Zucchi, P. and Zick, M. (2011) Membrane fusion catalyzed by a Rab, SNAREs, and SNARE chaperones is accompanied by enhanced permeability to small molecules and by lysis. Mol Biol Cell 22, 4635-4646.
33. Zick, M. and Wickner, W. (2016) Improved reconstitution of yeast vacuole fusion with physiological SNARE concentrations reveals an asymmetric Rab(GTP) requirement. Mol. Biol. of the Cell 27, 2590-2597.
34. Song, H., Torng, T., Orr, A., Brunger, A.T., and Wickner, W. (2021) Sec17/Sec18 can support membrane fusion without help from completion of SNARE pairing. eLife 2021; 10:e67578 DOI: 10.7554/eLife.67578.